News
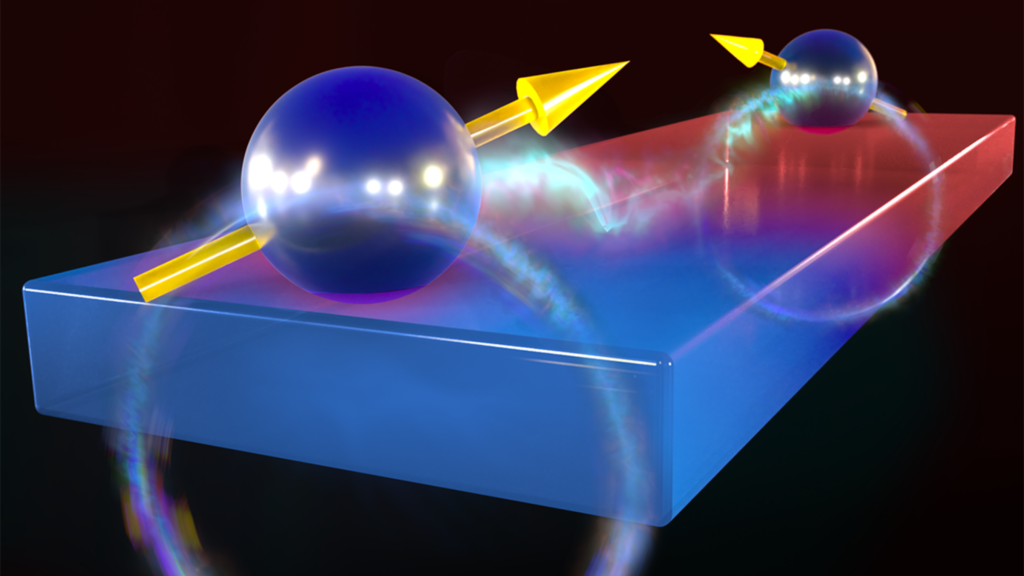
In novel quantum computer design, qubits use magnets to selectively communicate
Monday, January 29, 2024
Profs. Michael Flatte and Denis Candido collaborated on research that uses magnets to entangle qubits, the building blocks of quantum computers; the simple technique could unlock complex capabilities.
Faculty Specializing in this Area
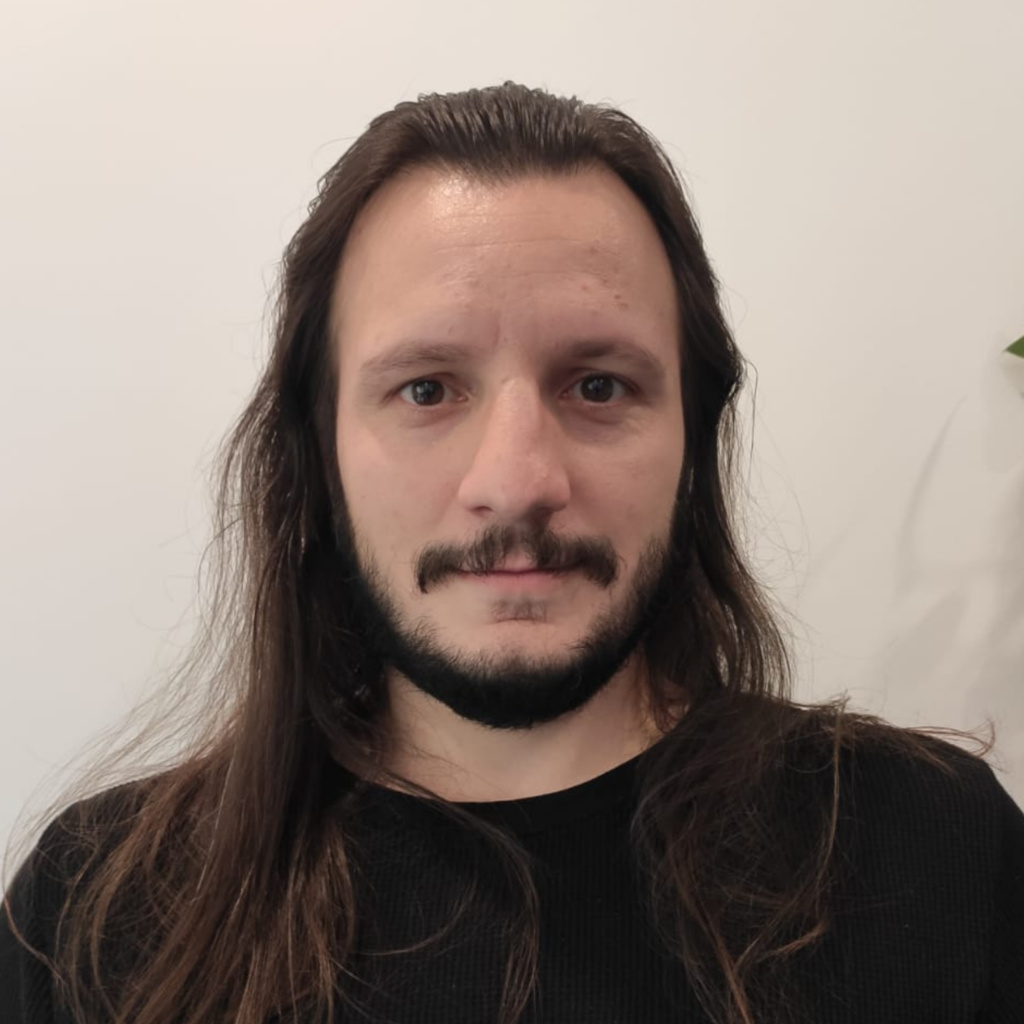
Denis R. Candido, Ph.D.
Title/Position
Assistant Professor

Michael E. Flatté, Ph.D.
Title/Position
Professor
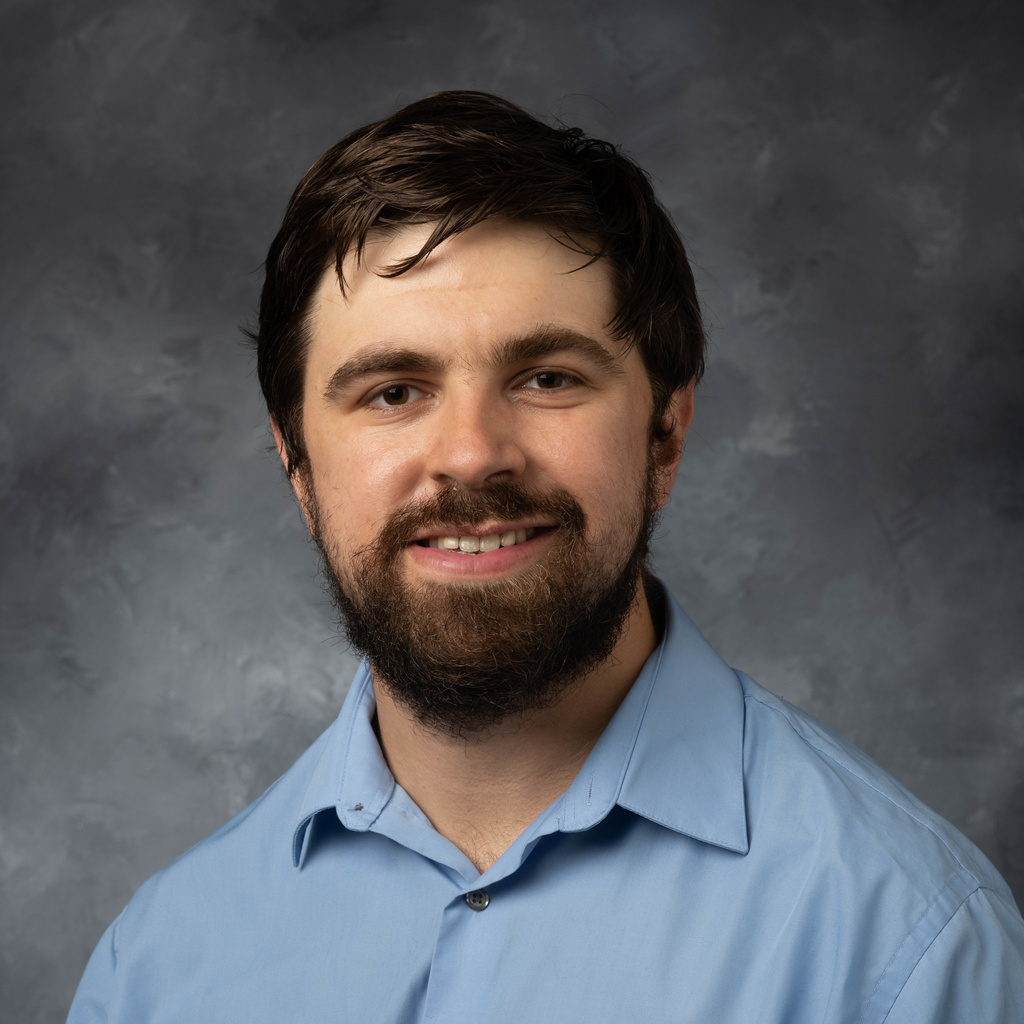
Thomas Folland, Ph.D.
Title/Position
Assistant Professor
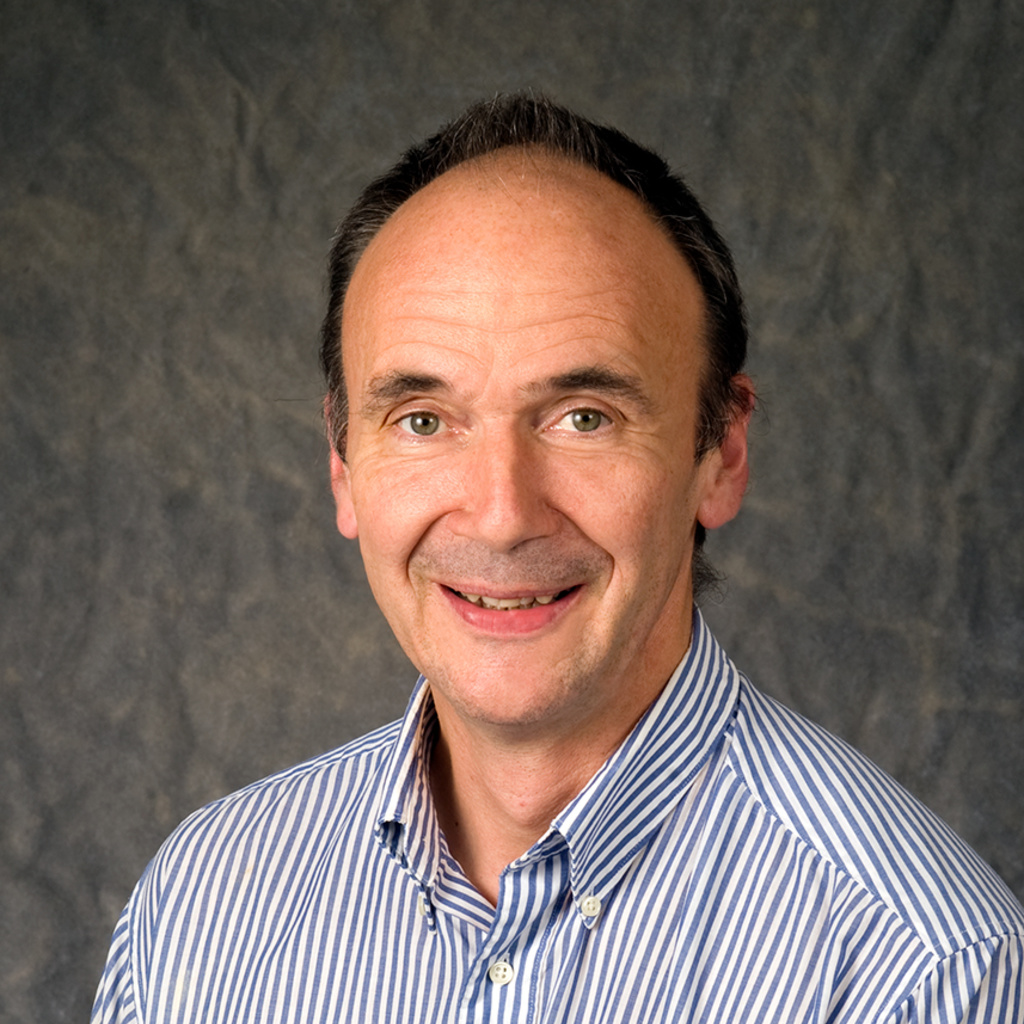
Yannick Meurice, Ph.D.
Title/Position
Professor
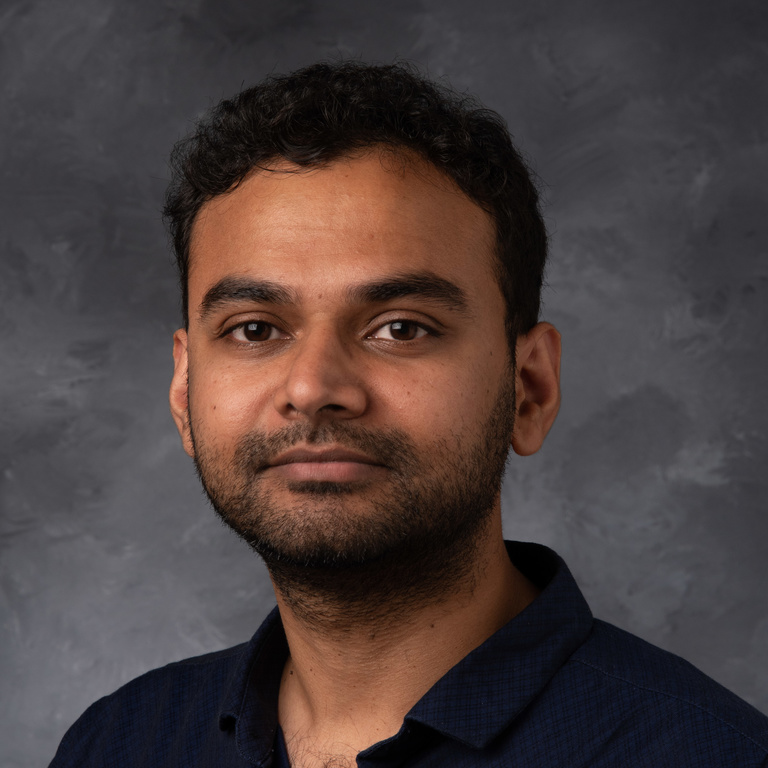
Ravitej Uppu, Ph.D.
Title/Position
Assistant Professor
Research Staff in this Area
There are currently no results to display.