Physics and Astronomy students presented 11 posters at the Fall Undergraduate Research Festival (FURF) Nov. 2 at the University Capitol Centre.
The Iowa Center for Research by Undergraduates hosts FURF, held in celebration and recognition of undergraduate researchers' contributions to research, scholarly, and creative work at the University of Iowa. It is a low-stress platform for undergraduate students to practice communicating their research, scholarly, and/or creative work to a broad audience. Presenters represented a broad range of sciences, arts, and humanities.
Aden Hageman and Jacob Andrews will be awarded $100 prizes for having the top Physics and Astronomy posters.
Physics and Astronomy students presenting posters are listed below with their faculty mentor, poster title, and abstract.

Jacob Andrews
Major: Physics
Graduation: Spring 2025
Mentor: Dr. Jane Nachtman
DUNE, Deep Learning for Neutrino Physics
The Deep Underground Neutrino Experiment (DUNE) seeks to understand neutrino oscillations by constructing neutrino detectors at Fermilab in Chicago and at SURC in Leads, South Dakota to observe how neutrinos change flavor during their flight. I develop deep learning algorithms to reconstruct the interactions we observe in the detector so that we can get the neutrino count at each detector and observe how the neutrinos changed across their flight.
Tom Bruner
Majors: Physics, Philosophy
Graduation: Spring 2023
Mentors: Drs. Jane Nachtman and Yasar Onel
Quality Control and Assembly Automation for Upgrades to the CMS Outer Tracker
At the Fermi National Accelerator Laboratory, I worked on automation processes and quality control procedures for the assembly of sensor modules going into the upgrade of the CMS Outer Tracker at the Large Hadron Collider at CERN.
Aditya Desai
Majors: Physics, Economics
Graduation: Spring 2024
Mentor: Dr. Thomas Folland
Characterizing the Optical Properties of Materials for Infrared Nanophotonics
Infrared light can be used to detect chemical compounds, observe the temperature of objects, and even engage in free-space optical communication. A key part of realizing technologies using infrared light is developing accurate models for the optical properties of the materials used. To do so, we can use Fourier-Transform Infrared (FTIR) Spectroscopy, where we quantify the light which is reflected and transmitted at different wavelengths. The measurements of reflectance can yield a variety of information about the optical properties of various materials, such as the concentration of additional impurities within the material and their crystal vibrations. These excitations can be used to excite surface light waves known as surface polaritons, which can pave the way toward designing new sensor technologies. For a variety of conventional materials, these properties have been identified; however, many new and exotic materials, various optical properties remain unknown and must be characterized. To characterize the optical properties of unknown materials, it is necessary to compare experimental data to data projected by a computer model based on various parameters, such as the thickness of the material, angle of incidence of the light, polarization of the light, and inherent properties of the material. By varying the parameters until the computer model’s projection closely matches the experimental data, it is possible to identify what the inherent properties of the material are. In my project I created a fitting algorithm within the model which adjusted the parameters within the model representing the inherent properties of the material until the projection most accurately matched the experimental data, and from that was able to characterize the inherent properties of the material. I validated the process by checking it with conventional materials such as sapphire (Al2O3) and silicon, before applying it to modified materials such as doped silicon carbide (SiC-4H) to identify and characterize its unknown properties. Overall, these results can be applied in future research to characterize more difficult and complex materials, such as low symmetry crystals, which are of interest to developing future technologies.
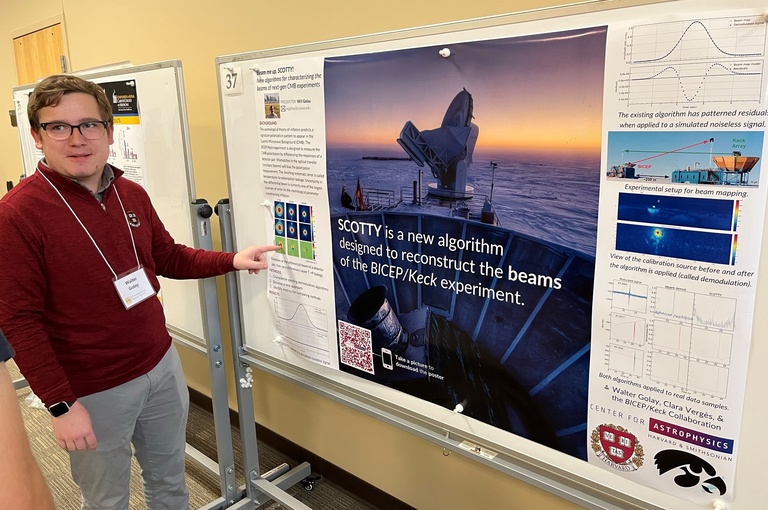
Walter Golay
Majors: Astronomy, Physics
Graduation: Spring 2023
Mentor: Dr. Robert Mutel
Beam me up, SCOTTY! New algorithms for characterizing the beams of next-generation CMB experiments
The latest cosmologies predict inflation in the early universe imprinted a signature polarization pattern in the cosmic microwave background (CMB). The BICEP/Keck collaboration aims to detect this unique pattern to constrain inflation models. However, precision CMB measurements such as those carried out by BICEP/Keck telescopes rely on a thorough understanding of instrumental systematics. For example, determining the differential beam between pairs of orthogonal detectors is essential to mitigating the effects of temperature-to-polarization leakage, a significant source of systematic error. The first step in characterizing the beams is the demodulation of a signal generated by observing a ground-based chopped source. Here we present a summary of previously implemented demodulation techniques and evaluate a new approach called SCOTTY (Systematic Cleaning of TimesTreams Yielding beams). We demonstrate that the existing time-domain kernel algorithm called square_demod has systematic, patterned errors intrinsic to the algorithm. SCOTTY circumvents this problem by employing the convolution theorem to perform the demodulation in Fourier space. This approach has the unique benefit of separating the down-sampling of the signal from the demodulation and is not limited to a specific de-convolutional algorithm. We predict that future developments of advanced adaptive kernel weighting schemes and de-convolutional algorithms will improve the benchmarks of each algorithm, respectively.

Mary Haag
Majors: Astronomy, Physics
Graduation: Spring 2025
Mentors: Drs. Jane Nachtman and Yasar Onel
Cooling System for CMS Barrel Timing Layer Upgrade
The University of Iowa CMS group is working on the Barrel Timing Layer cooling system in preparation for the LHC Run 4 at CMS. A prototype CO2 cooling system was developed by Iowa engineers and tested at Fermilab. Simulation studies using the FLUENT software were performed to understand the observed behavior, but limited by input parameter models. The fluid properties were studied to improve the input models for the temperature dependence of the CO2 viscosity using existing literature. The results of these studies and future prospects will be discussed.
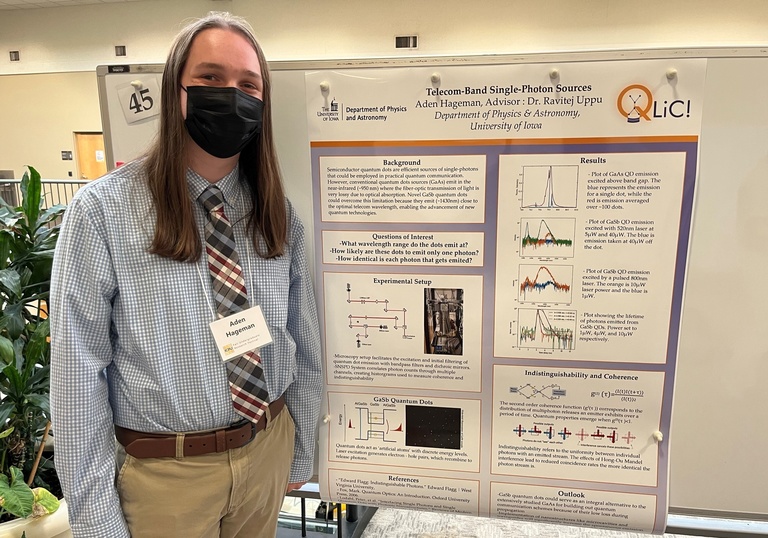
Aden Hageman
Major: Physics
Graduation: Spring 2024
Mentor: Dr. Ravitej Uppu
Telecom-band single-photon sources
Semiconductor quantum dots are efficient sources of single photons that could be employed in practical quantum communication. However, conventional quantum dots sources emit in the near-infrared (~950 nm) where the fiber-optic transmission of light is very lossy due to optical absorption. Here, we demonstrate novel quantum dots in gallium antimonide that emit (~1430 nm) near the optimal telecom band, which could therefore enable long-distance quantum communication. Through detailed spectroscopy and photon state characterization, we quantify the quality and intrinsic efficiency of these single-photon sources. Moving forward, we will embed the quantum dots in nanophotonic structures to boost the collection efficiency of photons into an optical fiber.
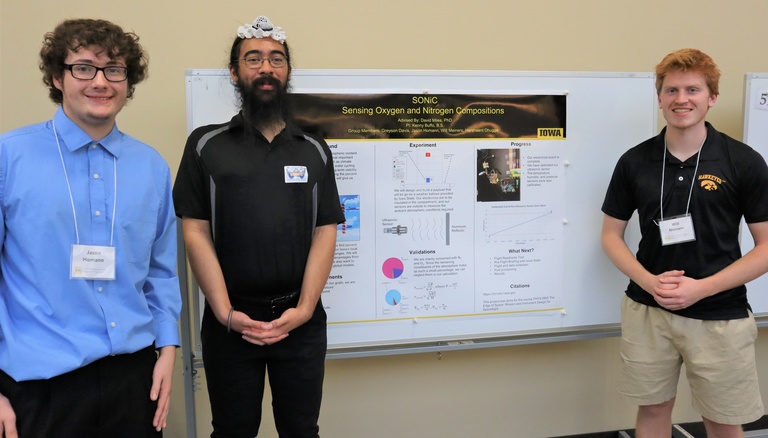
Jason Homann; Will Meiners
Majors: Physics, Astronomy; Physics, Astronomy
Graduation: Spring 2025; Spring 2025
Mentor: Dr. David Miles
Sensing Oxygen and Nitrogen Composition (SONiC)
Accurate measurements of local atmospheric content enable the study of a number of geophysically important topics, including climate change, heat transfer, and water cycling, which affect the long-term viability for life on earth. In an effort to address this need for geographically relevant information regarding Iowa's atmospheric profile, accurate measurements of the relative abundances of diatomic nitrogen (N2) and oxygen (O2) gas are required. We propose a mission to fabricate an experimental payload instrument capable of measuring the relative abundances of these gases within Iowa's atmosphere. This mission seeks to compare the global estimations for N2 and O2 abundances to that locally of Iowa. This will be achieved by measuring how changes in N2 and O2 abundances affect the speed of sound as a function of altitude above Iowa. Additionally, we seek to model how the abundance of water vapor, an atmospheric trace element, affects the presence of N2 and O2. The instrument will be delivered on a high-altitude meteorological balloon to be launched from central Iowa, and will monitor sound propagation, altitude, pressure, temperature, and humidity in order to meet these science goals. We intend to complete an analysis that relates these observable parameters to the abundances of N2 and O2, as well as compare these abundances to those reported globally.
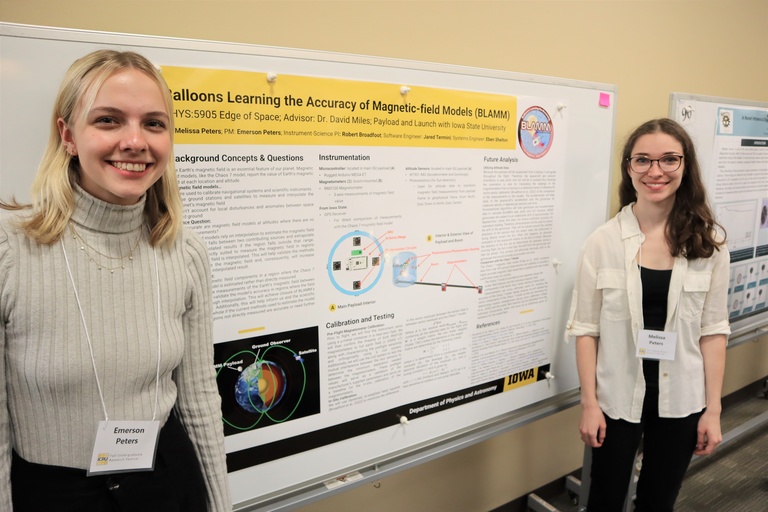
Melissa Peters; Emerson Peters
Majors: Physics, Mathematics; Physics
Graduation: Spring 2023; Spring 2025
Mentor: Dr. David Miles
Balloons Learning the Accuracy of Magnetic Field Models (BLAMM)
Earth’s magnetic field is essential for human science and activities. Magnetic field models, like the Chaos-7 model, provide field values for each location around the Earth. Model values are used to calibrate scientific sensors and monitor their interactions with Earth’s magnetic field. Flights and experiments reference model values to properly shield their instruments from magnetic interactions with Earth. These model values allow for calibration of compasses, GPS, and consumer navigational systems. However, magnetic field models have their downfalls: ground stations and space satellites measure field values and, by interpolating these data points, estimate the field values at each location in between measurements. There are no direct measurements between these areas, and models do not consider local disturbances and anomalies. How accurate are magnetic field models at altitudes where there are no direct measurements? Balloons Learning the Accuracy of Magnetic-field Models (BLAMM) aims to verify estimated values of magnetic field models. BLAMM’s goal is to take direct measurements at up to 30 km in altitude to explore if the interpolated values between space and ground are accurate. BLAMM’s discoveries will act as a step towards validating model estimates, even with just a single data point from neither ground nor space.
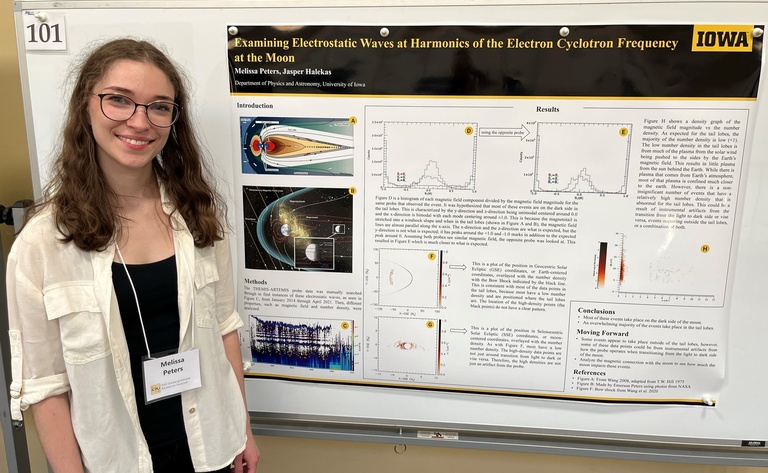
Melissa Peters
Majors: Physics, Math
Graduation: Spring 2023
Mentor: Dr. Jasper Halekas
Examining Electrostatic Waves at Harmonics of the Electron Cyclotron Frequency at the Moon
The two THEMIS-ARTEMIS probes have provided a wealth of data on the plasma environment of the Moon, with temporal coverage extending from 2011 to the present. The Moon interacts with plasma in the solar wind and terrestrial magnetosphere, acting as a sink for ambient plasma, as well as a source of charged particles through processes such as photoemission and secondary emission. These interactions simulate a wide variety of plasma instabilities around the Moon, which result in electromagnetic and electrostatic signatures across a broad range of frequencies. One commonly observed signature consists of electrostatic waves with emission at harmonics of the electron cyclotron frequency. These waves are observed most frequently in the terrestrial magnetotail lobes and in the shadow of the Moon but can also occur in other locations. We survey ARTEMIS data from the entire mission to determine when and where these waves occur. We analyze the locations of the probes with respect to the Moon and the Earth when they detect these waves, as well as the local plasma properties, in order to search for correlations that would provide possible explanations for the causes of these phenomena.
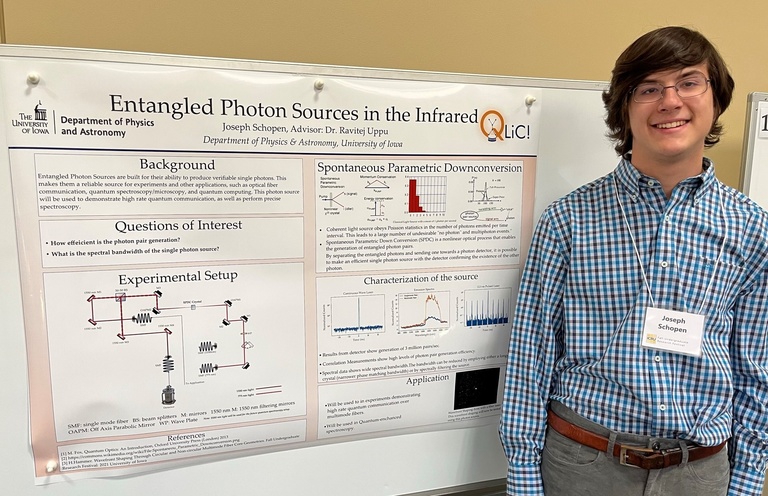
Joseph Schopen
Majors: Physics, Astronomy
Graduation: Spring 2024
Mentor: Dr. Ravitej Uppu
Entangled Photon Sources in the Infrared
Spontaneous Parametric Down Conversion (SPDC) is a nonlinear optical process that enables the generation of entangled photon pairs. I built a versatile photon source that can be operated to either generate two telecom band photons for implementing quantum communication or can generate frequency-entangled photons in the mid-infrared (2200 nm - 3500 nm) for applications in quantum-enhanced spectroscopy. Here, I report the spectral measurements of the emitted photons as well as the second-order correlation measurements that quantifies the pair generation efficiency. We demonstrate generation of >3 million pairs/sec (~1 pW) highlighting the brightness of our source. The immediate application of the source is towards demonstration high-rate quantum communication over multimode fibers.

Zach Vig
Majors: Physics, Geology
Graduation: Fall 2022
Mentor: Dr. Emily Finzel (Earth and Environmental Sciences)
Quantifying geochemical provenance of the Beaverhead group in southwestern Montana using unsupervised machine learning on a sparse dataset
A solid understanding of both the depositional age and provenance of the Late Cretaceous-Early Cenozoic Beaverhead Group is vital in analyzing the nature and timing of flat-slab subduction during the Laramide orogeny. This study aims to use whole rock geochemical signatures to connect Paleozoic carbonate source rocks to those of the carbonate conglomerate clasts from the Beaverhead Group. By using unsupervised machine learning, we hope to develop new methods for geochemical provenance analysis on carbonates, better constrain the provenance and transport pathways for the Beaverhead Group and, finally, characterize the extensive shallow marine carbonates that exist in much of Southwestern Montana. Preliminary results have shown that source rock carbonates are able to be distinguished in a geologically meaningful way and that their connection to the provenance of the Beaverhead Group mostly supports previous geological interpretations.